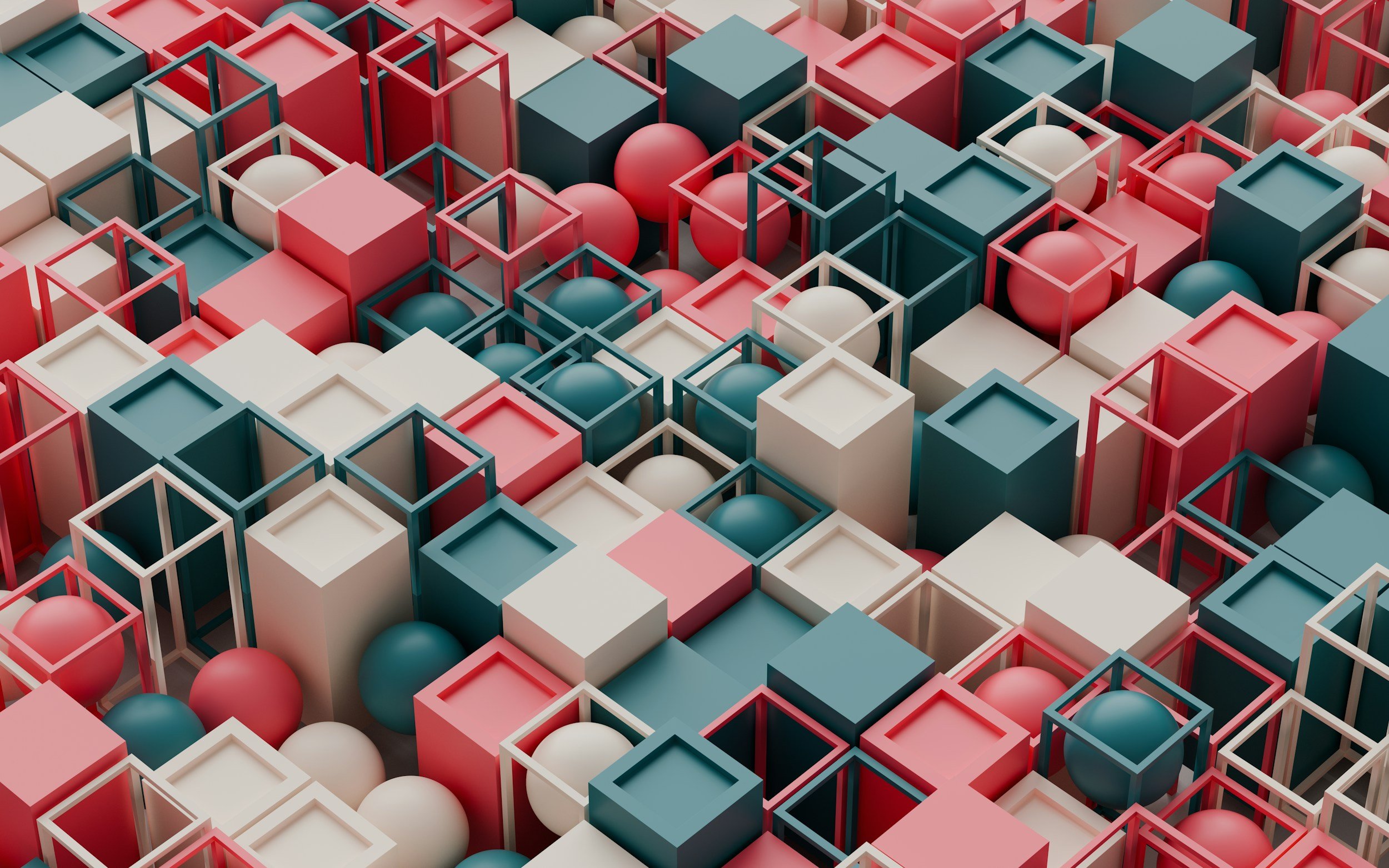
RESEARCH
How cells control their protein content: an introduction
Cellular protein levels are determined by both synthesis and degradation. In eukaryotic cells, the Ubiquitin-Proteasome System controls most selective protein breakdown, involved in almost all aspects of cellular activities. Misregulation of protein degradation is widely associated with human pathology, such as cancer, dementia, muscle dystrophy, autoimmunity, aging and etc.
Most proteins can be ubiquitylated, which is catalyzed by ~600 ubiquitylation pathways covalently attaching ubiquitin, a 76-amino-acid small protein, to lysine residues on the targets and ubiquitin itself. The resulting complex patterns of ubiquitin form an enigmatic “ubiquitin code” and dictate the consequence of this modification. Various effector proteins, including the proteasome, recognize this code and execute its instructions to regulate the rate and pathway of protein degradation.
Our research focuses on three major questions:
What is the ubiquitin code? and how does the proteasome recognize the ubiquitylation pattern on protein targets to regulate the rate and outcome of target degradation?
Are there unknown regulators of the ubiquitin-proteasome system? and how do they regulate cellular activities?
How can we effectively control protein stability, for treating diseases?
To understand how the proteasome and other protein machines work requires knowledge in their dynamical behaviors, which is a main research focus in the post-structure era. So far, no effective approach is available to directly probe their structural dynamics. Computer simulation using conventional methods in a biologically-relevant time scale is still impractical (longer than 1000 years for the proteasome!).
In a ground-breaking study, we developed a novel approach to enable the simulation of proteasome’s structural dynamics, using its energy landscape that was determined empirically. We find that predictions of the simulation are widely consistent with experimental observations in this and previous studies. This consistency validates our approach. This energy-landscape-based simulation rationalizes previous results that appear contradictory and provides mechanistic explanation for, particularly, how different subunits on the proteasome coordinate in degrading a protein target. We find that the proteasome has a unique stable, or energy-minimum, conformation regardless of its chemical status. This feature allows unambiguous collective conformational changes of proteasome’s subunits upon stimulation by chemical energy.
How the Shredder Works: Structures and Dynamics of the 26S Proteasome
At the center of eukaryotic protein degradation is the 26S proteasome, an elegant protein machine assembled from 33 subunits. To degrade a protein target, the 26S proteasome recognizes the ubiquitin configuration on the target, engages it, progressively unfolds and translocates the target molecule into the central chamber for proteolysis.
To understand how the proteasome and other protein machines work requires knowledge in their dynamical behaviors, which is a main research focus in the post-structure era. So far, no effective approach is available to directly probe their structural dynamics. Computer simulation using conventional methods in a biologically-relevant time scale is still impractical (longer than 1000 years for the proteasome!).
In a ground-breaking study, we developed a novel approach to enable the simulation of proteasome’s structural dynamics, using its energy landscape that was determined empirically. We find that predictions of the simulation are widely consistent with experimental observations in this and previous studies. This consistency validates our approach. This energy-landscape-based simulation rationalizes previous results that appear contradictory and provides mechanistic explanation for, particularly, how different subunits on the proteasome coordinate in degrading a protein target. We find that the proteasome has a unique stable, or energy-minimum, conformation regardless of its chemical status. This feature allows unambiguous collective conformational changes of proteasome’s subunits upon stimulation by chemical energy.
Related publications:
Shuobing Chen*, Jiayi Wu*, Ying Lu*, Yong-Bei Ma, Byung-hoon Lee, Zhou Yu, Qi Ouyang, Daniel J. Finley, Marc W. Kirschner, and Youdong Mao (2016). Structural Basis for Dynamic Regulation of The Human 26S Proteasome. PNAS Nov. 15; 113(46): 12991-12996. *equal contribution
Ying Lu*, Jiayi Wu*, Shuangwu Sun, Yong-Bei Ma, Qi Ouyang, Daniel Finley, Marc W. Kirschner, Youdong Mao (2017). Conformational Landscape of the p28-bound Human Proteasome Regulatory Particle. Molecular Cell Jun 26; S1097-2765(17). *equal contribution
Yanan Zhu, Wei Li Wang, Daqi Yu, Qi Ouyang, Ying Lu*, Youdong Mao* (2018). Structural mechanism for nucleotide-driven remodeling of the AAA-ATPase unfoldase in the activated human 26S proteasome. Nature Communication Apr 10;9(1):1360. * Co-corresponding author
Yuanchen Dong, Shuwen Zhang, Zhaolong Wu, Xuemei Li, Weili Wang, Yanan Zhu, Svetla Stoilova-McPhie, Ying Lu*, Daniel Finley, Youdong Mao (2019). Cryo-EM structures and dynamics of substrate-engaged human 26S proteasome. Nature. 565(7737):49-55.
Deciphering the Death Code of Protein Molecules
Related publications:
Ying Lu, Byung-hoon Lee, Randall King, Daniel Finley and Marc W. Kirschner (2015). Substrate Degradation by the Proteasome: A Single-Molecule Kinetic Analysis. Science, 348:1250834.
Ying Lu, Weiping Wang, and Marc W. Kirschner (2015). Specificity of the Anaphase-Promoting Complex: A Single-Molecule Study. Science 348:1248737.
Byung-Hoon Lee, Ying Lu, Miguel A. Prado, Yuan Shi, Shuangwu Sun, Suzanne Elsasser, Marc W. Kirschner, Steven P. Gygi, Randall W. King, and Daniel Finley (2016). USP14 Deubiquitinates Proteasome-bound Substrates that are Ubiquitinated at Multiple Sites. Nature 532:398-401.
Nicholas G. Brown, Ryan VanderLinden, Edmond R. Watson, Florian Weissmann, Alban Ordureau, Kuen-Phon Wu, Wei Zhang, Shanshan Yu, Joseph S. Harrison, Renping Q. Coudevylle, Iain F. Davidson, Ying Lu, Prakash Dube, Michael R. Brunner, Christy R. R. Grace, Darcie J. Miller, Marc A. Jarvis, Masaya Yamaguchi, Peter Y. Mercredi, Sachdev S. Sidhu, Brian Kuhlman, Marc W. Kirschner, J. Wade Harper, Jan-Michael Peters, Holger Stark, Brenda A. Schulman (2016). Dual RING E3 Architectures for Substrate Polyubiquitination Visualized for Anaphase Promoting Complex/Cyclosome. Cell 165(6);1440-53.
Jason Hon, and Ying Lu* (2018). Single-molecule methods for measuring ubiquitination and protein stability. Methods in Enzymology 619: 225-247. *corresponding author *review article
Hundreds of ubiquitylation pathways conjugate complex patterns of ubiquitins on numerous protein substrates to alter their properties, such as stability, localization, activity and interaction partners. Due to the complexity and experimental challenges, we still do not understand the rules by which the proteasome recognizes this ubiquitin code and commits the destined substrate into an irreversible degradation process.
In previous studies, we introduced chemical methods for generating substrates with defined ubiquitin configurations, and developed single-molecule kinetics approaches to illuminate the transient reaction steps and reaction intermediates. We also developed high-throughput methods to identify the ubiquitin configurations on physiological substrates. Powered by these novel technologies, we aim to understand how the ubiquitylation patterns on substrates, through interacting with the multitude of ubiquitin receptors on the proteasome, control the key steps in proteasomal degradation to regulate protein stability.
The Role of Protein Degradation System in Neurodegenerative Diseases
Cellular surveillance system identifies misfolded proteins and tags them for proteasomal degradation. Accumulation of misfolded and aggregated proteins compromises the ubiquitin-proteasome system and may underlie the age-dependent decline of proteasome activity, which progressively leads to neurodegenerative diseases. Activation of the proteasome activity using genetic and pharmacological approaches has been shown to ameliorate neurodegenerative phenotypes in several animal models of neurodegeneration.
Using biochemical and genetic screening, we identified novel regulators of the protein degradation system that can effectively accelerate cellular protein breakdown. We aim to understand their biological mechanisms and how their regulation may be involved in neurodegeneration. We hope our study will lead to novel therapeutic approaches to target neurodegenerative diseases.
Developing Chemical Modulators of Protein Degradation
The Ubiquitin-Proteasome System is a popular drug target. Proteasome inhibitors have been widely applied in treating cancers and autoimmune diseases, while activators of the proteasome have shown preliminary efficacy in animal models of neurodegenerative diseases. The recent development of Proteolysis Targeting Chimera (PROTAC) compounds enables selective degradation of protein-of-interest and has triggered strong enthusiasm in targeting multiple diseases.
Widely-used proteasome reporters detect the 20S activity which is not closely associated with proteasome’s primary function of degrading ubiquitylated proteins. In a previous study, we developed a novel reporter system that can accurately measure the ubiquitin-dependent activity of the 26S proteasome both in vitro and in vivo. We developed drug screening platforms based on these reporters to explore the pharmacological space for modulating the proteasomal activities. In an unpublished study, we identified novel modulators of the ubiquitin-proteasome system which may boost the efficacy of the proteolysis-targeting drugs.